By Amir Jafari.
An understanding of snap loads is crucial for the safe, sustainable operation of floating offshore wind turbines (FOWTs). Not least because, as yet, there are no standardised design guidelines for mitigating snap loads in FOWTs.
Here’s a quick introduction to snap loads: what they are, why they are important and how calculating them correctly can enhance the safety and profitability of FOWT developments.
Offshore wind and the function of mooring lines
The name “floating offshore wind turbine” suggests a degree of freedom and mobility that isn’t quite matched by reality. FOWTs must be anchored to the seabed – and rely on a station-keeping system to maintain their position within a designated area, allowing their connection to the electrical grid.
To stay connected to the seabed, FOWTs commonly employ three types of mooring system: catenary, taut (semi-taut) and tendons. Furthermore, a single-point mooring system is employed as a weathervane system for some specific platforms.
The design of mooring lines for FOWTs
In floating structures, the motions of the turbine platform and the tension in mooring lines are linked: restoring forces within the mooring system oppose destabilising loads to keep the platform in place.
The design of mooring lines for FOWT platforms is typically based on experience gained from the oil and gas industry. Yet there are significant differences in the FOWT environment – such as operating water depth (typically shallower than oil and gas), dynamic response characteristics, space clearance, wake effect and overall system scale (dimensions and mass).
The design of mooring lines is not something to be taken lightly. The dynamic nature of the marine environment presents a substantial threat to floating platforms when a mooring failure occurs. Between 2001 and 2011 there were 21 incidents involving floating offshore oil and gas production vessels, of which at least 8 involved the failure of multiple lines. Some of these incidents even led to the drifting of floating units. Data from 2014 reveal that roughly 34 semi-submersible platforms in the North Sea experienced three mooring failures every two years, primarily due to problems with mooring lines (Xu et al, 2023).
As yet, there haven’t been any publicly reported instances of mooring faults involving FOWTs. But the potential ramifications are huge. Unmoored turbines could drift into other structures or capsize. That could cause millions of pounds worth of damage. That’s why it’s so important to understand the forces that act on the mooring lines – in particular, snap loads.
An introduction to snap loads
Snap loads are one of the main factors contributing to the failure of mooring lines. They are defined by an abrupt shift in tension magnitude travelling along the mooring line or cables. These loads have the potential to induce shocks on the line material, significantly diminishing its fatigue life.
A snap event is defined by a rapid surge in tension, over a short duration, as a mooring line re-engages instantly after a slack condition. This typically occurs when FOWTs experience large wave and wind-stimulated motions, but can potentially happen across a range of sea states (HSU et al, 2018).
In accordance with Palm et al (2017), two additional mechanisms contribute to the generation of snap loads in mooring lines. Shockwaves can occur due to nonlinear material response, particularly common in materials comprising synthetic fibre ropes. Snap loads can also occur when the touchdown point velocity of a chain exceeds the wave speed in the transverse direction of the line. This is especially noticeable in slack catenary moorings (Palm et al, 2017).
Design guidelines for snap loads
There are two DNV documents in which snap loads are discussed. DNV-OS-H206 addresses the loadout, transport and installation of subsea objects, while DNV-RP-H103 focuses on the modelling and analysis of marine operations.
However, the recommendations outlined in DNV standards are primarily defined for subsea lifting operations and other similar operations. There are currently no specific criteria for FOWTs regarding snap loads. Hsu et al (2018) revised the rules from DNV (2011) and described the slack condition as 10% of the mooring line’s pretension or less. A snap event begins after the tension value drops below this minimum threshold, and lasts until the tension spikes to a value greater than pretension.
The total line tension (Ftotal) is the linear sum of static/pretension (Fst) and dynamic tensions (Fdyn):
Ftotal=Fdyn+ Fst
The snap event happens when the dynamic force exceeds 90% of the static load:
Fdyn>0.9 Fst
Snap load simulations for FOWTs
To simulate snap events in a floating offshore wind turbine, the NREL 5MW semisubmersible (Robertson et al, 2014) was utilised in OrcaFlex. The chosen configuration involved a shallow water depth of 50m with a hybrid mooring system comprising both chain and fibre rope. Three catenary mooring lines, consisting of one windward and two leeward lines, each with a length of 390m, were employed. The model underwent a two-hour simulation under conditions of 2.9 m significant wave height (Hs) and 7.7 s zero-crossing wave period (Tz), at a rated wind speed of NREL 5MW wind turbine, 11.4 m/s.
With a relatively low pretension of 250 kN for the windward line, the maximum tension experienced reached 2700 kN. Utilising the equations mentioned above, the slack condition was determined to be 25 kN. The line experienced a total of 11 snap events during the simulation, ranging from 950 kN to 1600 kN.
You can observe the mooring tension time series from 1160 seconds to 1300 seconds in the figure below. The red line indicates threshold, the blue circles demonstrate the slack event and the red circles show the snap load.
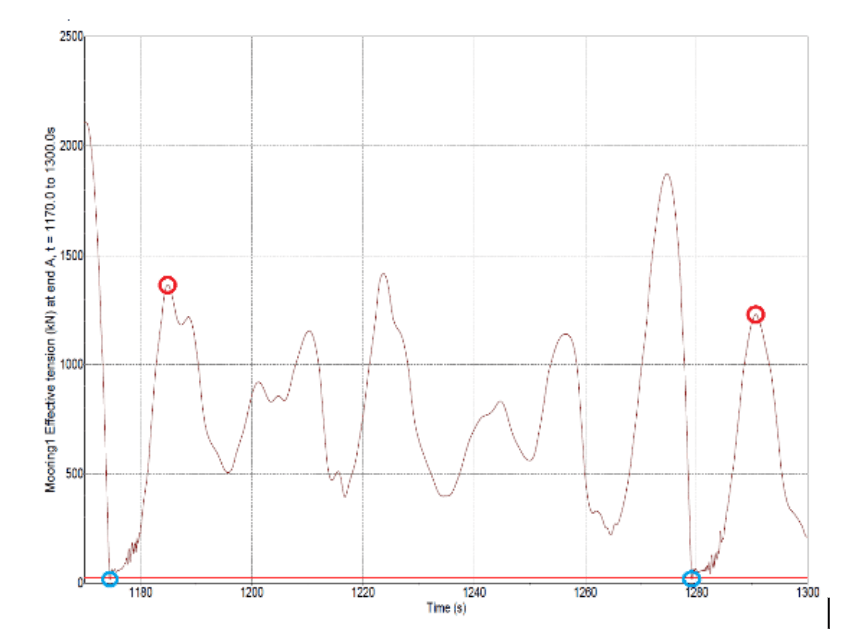
The primary factors influencing mooring line dynamics in shallow water are line stretching and sensitivity to wave excitation. In instances where a mooring line is relatively short, it lacks sufficient elongation for axial stretching, leading to the occurrence of the frequently observed snap condition (HSU et al, 2018).
Snap loads: material choice and mitigation strategies
Despite the utilisation of fibre ropes resulting in snap loads in the above simulation, it’s essential to recognise that fibre ropes are generally chosen to prevent such occurrences in mooring lines. This selection is based on their inherent characteristics, including high elasticity and fatigue resistance, which are typically effective in mitigating snap loads.
The mooring line design for FOWTs requires a balance, optimising platform surge, pretension and peak tension along the line, while actively avoiding vertical loads on the anchors. When facing snap loads, it’s imperative to strike a balance – addressing both the mitigation of snap load risks and the avoidance of excessive pretension.
Clamp weights are increasingly employed in mooring line configurations to address snap loads. This process involves contributing to inertia by adding weights along the lines. This extra weight helps mitigate sudden dynamic loads on the mooring line.
There are also ongoing industry initiatives to tackle the issue as illustrated below. This device features a buoyant end and a weighted end connected within a mooring line, primarily positioned vertically. As the turbine platform undergoes movement, the device rotates, extending the overall length of the mooring line. The combined effect of weight and buoyancy generates a restoring force, bringing the device back to its nominal vertical position (Dublin Offshore Website).
In conclusion …
Despite the available research dedicated to snap loads, there is a need for standardised protocols to offer more precise guidance. Establishing specific guidelines for floating offshore wind turbines is crucial, ensuring that the challenge of snap loads is thoroughly considered during the concept design phase of mooring lines.
How can we help?
Here at Empire Engineering, we are highly experienced with the design and implementation of complex offshore wind developments. Take a look at our services to see how we could assist your project.
Empire specialists can effectively and efficiently assist with your offshore wind project. To find out more, please get in touch with the team at Empire Engineering.